Water & Land Resource Management
The study of water and land resource management includes the hydrology and land degradation processes within a particular landscape context. It requires the application of sustainable water and land resource management programs in agriculture, forests, grasslands, recreation areas and urban zones. It requires the development of practical land management ideas and implementation for conservation purposes of land and water resources now and into the future.
What is Marginal Land? Government Departments, Real Estate Agents and Conservationists all have different concepts of what rates a piece of land marginal. Often marginal land is dictated by the individuals perspective of what money can be made from the land at that moment in time without any real thought of the future prospects of the lands worth as agricultural land or environmental preservation for the future or how it fits into the web of life. Marginal lands are often in areas that have density population pressures despite their real value in the ecological sense or the fragility of wildlife corridors or the land being exposed to erosion.
In Coffs Harbour the Local Council described my land as “marginal” and as a consequence was not worthy of farming. Land which has little immediate usefulness, because of problems associated with access, water control, topography, environmental restrictions or other factors deems it to be rated marginal. Strange as after 8 years of building the soil up; organically, the farm was transformed into the most profitable farm in the district yet it was rated as marginal. I often wonder what we could have produced if the land was rated as good “A” grade land for farming.
To call something “marginal” means it is not very good. Farmers have their own way to define marginal land: It is the last to be planted under good conditions, and the first to be avoided under poor conditions.
Low quality, infertile soils are not the only reason land is considered to be marginal. It might be in an area where rainfall is limited, a hillside might rise too steeply, chemicals have been used in the past or are at naturally high levels within the soil, (Our farm had areas that had high Boron and the whole farm was affected initially with high levels aluminum causing very low PH levels), salt affects the well being of the soil or even exposure to environmental issues like wind or regular flooding.
Marginal land usually has little or no potential for profit, and often has poor soil or other undesirable characteristics. This land is often located at the edge of deserts or other desolate areas.
Marginal land is usually fairly low in value. It is marked by its inability to produce crops including grass and trees of any kind or otherwise yield a profit.
Most marginal lands are placed in dispute between short term human well being and the need for environmental conservation. This conflict is particularly noticeable in the fields of water resource management, land degradation management, deforestation and sustainable development.
While most valuable conservation areas are locked up in National Parks many areas that constitute valuable wildlife corridors are still at the mercy of mercenaries out to make a quick dollar. Wildlife corridors are often the strips of marginal lands that exist between 2 or 3 declared National Parks and are not directly used by the wildlife for their existence. Without these corridors the value of the National Parks is lessened.
The problems of water resource management and environmental conservation on marginal lands are generally accepted as being four fold.
* Conflict exists and is growing between the limited supply and demand of this valuable commodity. It is a conflict created by rapid human population expansion, agricultural interests, wildlife and economic development.
* Conflict is highlighted between upstream users and downstream users within the whole watershed. This has been exemplified with the disagreements between cotton farmers on the upper Murrumbidgee in New South Wales and Queensland and the lower Murray River residents, especially the people in and around Adelaide in South Australia. Here politicians ride the political role of emotion with confrontation instead of dialogue and reason.
* These conflicts are further exasperated between those using the source efficiently like organic growers with the use of new technology like mulching and newly devised irrigation dispersal and institutions placing constraints on smaller stakeholders which deliver greater benefits to the institutions. These often look fair collectively but individually are far more beneficial to the institution.
* Trade offs in water resource allocation for human survival short term are at the cost of environmental survival long term. Governments have strong inclinations to place environmental conservation as a low priority.
Many people believe by simply diverting water from one water shed to another will solve the problem of a lack of supply. Diverting water from one watershed does not fulfil the solution but in fact enhances the problem by allowing more inefficiencies to develop in the receiver and adds to the water hydromancy problems of the supplier which may include higher levels of, salinity lower surrounding water tables, decreasing flows, pollution via lack of flushing, scouring in upper sections of the catchment and or deposition of alluvial matter higher up the river delta and changed environmental conditions. It would be far better to adjust land use systems, improve efficiencies of water use and apply new technology systems and methods. This latter method is more reliant on larger holdings and institutions are better equipped financially and technologically to improve greater efficiencies than smaller stakeholders.
Governments can be instrumental in subsidising framers to install trickle irrigation systems or microfilm jetting where fogs can be utilized to convert air moisture to droplets where appropriate.
Tree barriers are needed to control water erosion and shade to rills, creeks and streams to prevent excess evaporation which will be a bigger problem as Global waring entrenches itself otherwise more marginal lands will develop.
The over withdrawal of underground water from bores and wells is also contributing to salinity problems and declining water tables or supply. Monitoring of the water table at the Roe Creek bore field which supplies potable water to Alice Springs has dropped 70 metres since pumping began in the 1970s. Presently it is falling at a rate of 1meter to 1.5 meters a year.
Whatever the supply, solutions need to be found to bridge the widening gap between the supplier and the consumers so all consumers have an adequate supply without diminishing the supply and degrading the environment any further. The following 5 issues have to be met:
* Shift the supply to close the gap.
As most runoff of water for supply is from precipitation or snow melt increasing supply is very limited and dependant on short term and long term weather conditions. There is a small window of opportunity through the rediverting of tertiary treated sewage water which may include desalinated water after its final use and tertiary treatment in a sewage treatment plant.
Another possibility remains in funnelling excess unpolluted water during storms or excessive wet periods back underground to recharge ground water systems.
Another method which proved very useful in Brisbane was to redeploy the old rain water tanks. Roof water catchment can account for over 50mm of a family’s actual water usage even on a small 2,000 litre tank. These will fill every time a few millimetres of rain fall. Larger tanks will extend the benefits further into dry periods.
* Shift the demand to close the gap.
Shifting the demand is a lot easier than shifting the supply. Shifting the demand relies on every consumer decreasing their actual water usage or to reuse marginal water on site. The best method to reduce demand is to vastly improve the efficiency of its use. Low volume shower heads were the first real incentive to decrease the demand in Australia followed by half flush toilets. Reusing shower water is a great way to save water and dollars. We stand in a large plastic bowl when showering and place this water in a bucket for reuse in the garden the following day. 2 persons save 50 litres of water a day which is around 20,000 litres a year. Flushing the toilet after the last person uses it first thing in the morning saves another flush or another 3,000 litres a year. Reusing the grey water or water from the washing machine on the garden saves another 80 or more litres a wash or over 12000 litres a year for the average family doing 3 small washes a week. With a little more ingenuity you can easily save 25,000 litres a year. If every Australian family did this it would add up to an amazing 200,000 million litres a year or about 1,080,000 Olympic pools. Watering your plants at night will save over 50mm of the water that is used during daytime watering. 4 hours a week adds up to an incredible 2000 or more litres a week or over 100,000 litres a year. Now Australians can easily save another 200,000 Olympic Swimming Pools. Mulching the garden and replacing lawns with drought resistant natives could add another 200,000 Olympic Swimming Pools to the equation. If we all do our little bit the water conservation this ultimately saves in electricity which saves environment air pollution which helps reduce the impact on global warming. We will be a lot better off.
The utilization of technology both financially and economically is an area where governments and institutions both can find rewards. Marginal waters are not presently utilised enough in large businesses. Cooling water is one area that hot water could be stored and reused for washing or disinfecting. This would not only decrease electricity charges but also save water that is used in the power plants for cooling. Often marginal water is discharged into the sewage system or piped out to tailing dams to evaporate. A lost resource is one that is not well used or used to its full potential. A good example is the amount of sewage water that is tertiary treated and piped out into the ocean instead of being used in wetlands to filter back into the river system either upstream or slightly downstream from its original draw point.
We have already mentioned agriculture as a prime position to start the cost savings in reducing water demand. These include minimal input through correct irrigation techniques, storage systems should be deep with smaller surface areas, recycling water wherever possible, grow crops that require less water in your area or grow crops that are more dependant on seasonal rainfalls and can be planted to suit these wet periods, maintain all equipment and use modern technology wherever possible.
How much water is wasted waiting for it to pass from the source to the end user. It varies considerably but consider this. In our home we have a standby basin at the four exit points. Exit 1 in the kitchen uses 320milliliters. Exit 2 and three use 1130milliliters and 1290milliliters while exit 3 uses 1330millilitres. As we shower using the naval shower system this requires 4 uses or 4.52 litres a day. Washing the dishes requires 3 usages or 0.96 litres a day. We rarely use the hot water at the hand basin however the laundry may be used once a day or 4.52 litres. This equates in our home coincidently to 10 litters a day or 3,650 litres a year of good rainwater from the tank being reused. it is reused in washing clothes and other house hold activities.
Maintenance of pipes, pumps and storage vessels saves unnecessary water losses. A dripping tap wastes over 12 litres a day seven days a week 30 days a month, 365 days a year or 624 litres a year. Replacing the faucet ring or tightening the pie join proves that repairs save money and waste. A farm with several drips even along one pipe wastes thousands of litres a year.
* Increase the price of water so equilibriums are met.
Increasing the price of a commodity to force the equilibrium has been proven to be the most effective method of changing people’s habits. This has been especially evident in the behaviour of farmers who refuse to act, but respond immediately on price change policies. Technical choices should be presented to people at the same time or before to give them a physical choice of direction.
Bioe conomical modelling of projects has also been found to be affective in stimulating farmers respond to price changes.
* Rationing the supply of water to close the gap between supply and demand.
While it must be taken into account that the environment needs water as much as the farmers where the rain falls there is also a right of those downstream and adjacent to the streams and rivers have an equal quota to fresh clean water. Like the air we breathe it is a worldwide commodity that needs preservation for all. What needs to be considered is, do those upstream need to be compensated and if so how much and how should the compensation be paid?
Water needs rationed on the basis of needs of the individual, the needs to balance efficiency of the water utilization and equity principle and environmental conservation.
Any compensation needs to take these into consideration along with the economic recession caused by a decline in the volume or quality of the water and the ecological value of the water in question to both the environment and future tourism or other prospective future developments in the area.
The problems associated water resources management is how to develop a suitable mechanism for transaction for the rights for water use, how to measure the economic and ecological value of a water resource in the different areas that is seen to be fair to all parties with the present and future in mind and how to establish an institutional and organizational scheme so that all the players are dealt with fairly including the environment which many city people like the farmers consider vitally important to their existence.
* Introduce a transaction quota system that is fair to all consumers
including the environment.
Another method would be to make water transferable between farmers within a watershed. If this were to be adopted a farmer may be more content to allow their section or supply of water to be sold or transferred to another player else where. At Nana Glen we built a dam that held 4 years supply of water. This gave us water security but also provided the environment with a good flow after year one increasing the wildlife, until the new neighbours moved in and cleared the area along the creek banks destroying the habitat zone in place. This is an area where farmers are controlled but ignorant individuals are allowed to run rampant.
The microbiology of acid sulphate soils and sulphide sediments
Land and water management near the coast has been a disaster for over a century. The uncontrolled clearing, draining, exposure to coastal storms and canal estates have all contributed in creating the worst acid sulphate soils imaginable with very little controllable management being put in place until it is too late.
“Sea grasses” are usually associated with mud flats especially within enclosed bays or large flat shelves where Mangroves exist. They often grow on the ocean side where shallow water exists at low tides. The waters are oxygen rich while the soils are usually nutrient rich. These shallow intertidal zones are the staple grazing and foraging pastures in the diet of dugongs, green turtles and many small fish or fingerlings and provide a habitat for many smaller marine animals, some of which, like prawns and fish, are commercially important.
“They also stabilize sedimentary deposits, preventing scouring and assist in maintaining water clarity.
Acid sulphate soils and their associated sulphide sediments present a major hazard to sustainable farming, aquaculture, water quality and urban infrastructure along the coast. In the past these soils are heavily limed in order to neutralize the ‘leachate’ that is a public health hazard and extremely toxic to aquatic organisms. It may be more sustainable to exploit the soil microorganisms capable of sequestering metals to remediate these soils. Until recently, little was known about the microbiology and ecosystems of these environments. For soils they are strongly acidic at 3.5pH to 4pH and as far as an ecosystem is concerned correlate to the high end of where metal concentrations are expected and bio accessibility can be found. These environments have the potential to provide insights into how environmental conditions shape the micro biome that can be exploited for biotechnologies and biotechnologists alike.
The formation and management of acid sulphate soils:
Worldwide, the pressure for land to meet food security and urban development needs can lead to the disturbance of waterlogged soils containing iron-sulphide minerals. These minerals are oxidized to form acid sulphate soils; a toxic legacy of rain induced acidic and metalliferous ground waters that lead to the corrosion of infrastructure and the severe deterioration of water quality. The associated formation of mono sulphides in affected waterways is equally problematic, capable of de-oxygenating the aquatic ecosystem in seconds if disturbed.
Acid sulfate soils form wherever the following conditions are present.
Sulfate-reducing iron bacteria
These bacteria make pyrite crystals as a side effect of their metabolism. They can only survive when there is:
1. an anaerobic (oxygen depleted) habitat. This means the soil is saturated with water that becomes basically immobile. The lack of oxygen protects both the bacteria and the pyrite they create,
2. a supply of organic matter, which is in the form of decaying leaves from surrounding trees supply carbohydrates for the bacteria,
3. the presence of sulfur (in the form of sulfate SO42-) This supplies the bacteria the oxygen to respire’. the sulfate reducing bacteria uses the sulfate and produce hydrogen sulfide (H2S) the way animals use oxygen and produce carbon dioxide. Sulfate is a normal component of seawater, so acid sulfate soils commonly develop along the coast.
4. Iron, in its reduced form (Fe2+), reacts with the hydrogen sulfide (H2S) produced by the sulfate-reducing bacteria, to form pyrite (FeS2). As iron and sulpher are very common mineral elements, there is rarely a shortage for the bacteria.
Water movement
As pyrite forms, bicarbonate also forms from the same reaction. If it is not removed from the environment, the pyrite-forming reaction eventually slows and can stops the waters movement. Water movement (like tidal flushing of the system) will remove bicarbonate and leave the pyrite behind, allowing it to accumulate.
A typical stagnant, anaerobic, rill with copious vegetation coupled with an iron and sulphate enriched soil.
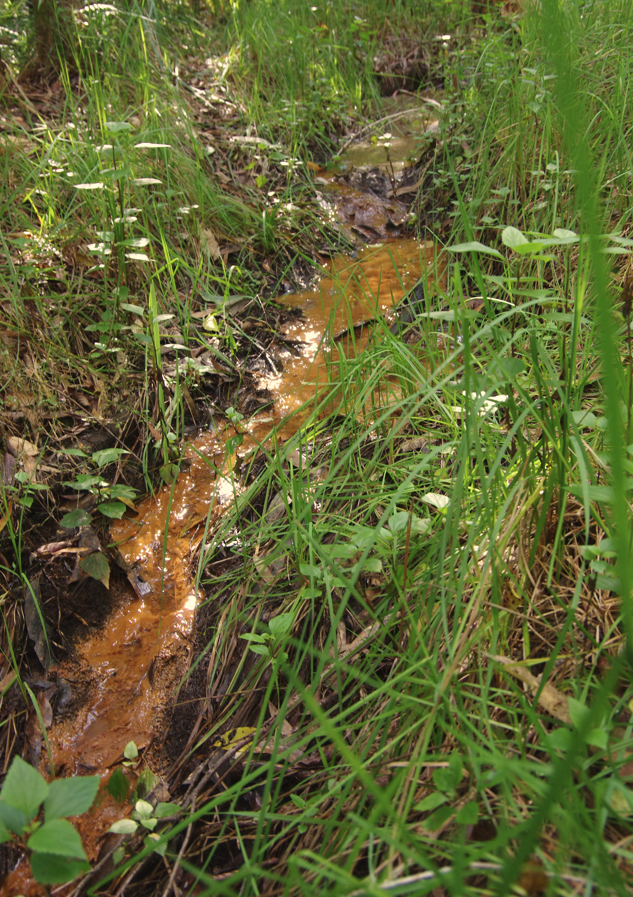
New acid sulphate soils form naturally in ephemeral swamps adjacent to wallums, especially mangrove forest areas and salt flats. Existing acid sulphate soils are commonly found where ancient mangrove forests and salt flats had existed in the past.
Current management and treatment practices of acid sulphate soils include minimizing the formation of acidity; neutralizing acidity and/or turning land back to wetlands. The ongoing cost of these disturbed environments in Queensland alone is extraordinary. “Overall, it has been estimated that the cost to industry of managing ASS in Queensland is approximately $189 million per annum.” – https://ozcoasts.org.au/indicators/coastal-issues/econ_cons_acid_sulfate_soils/
Up until recently, little was known about the microbial community in coastal acid sulphate soils. Nonetheless; rates of mineral transformation and mineral transformation products cannot be fully explained by abiotic processes, indicating that soil bacteria play an essential, but unknown role in element cycling in this environment. Furthermore, under biotic, redox cycling conditions, the rapid (days to months) transformation of meta-stable minerals to stable minerals, which concomitantly sequesters trace contaminants could be possible. Thus, understanding the microbial ecology, and particularly the identification of bacteria capable of iron-sulphur transformations, is an essential step towards exploiting the microbial community to remediate these environments.
Up until recently, little was known about the microbial community in coastal acid sulfate soils. Nonetheless; rates of mineral transformation2 and mineral transformation products3, 4 cannot be fully explained by abiotic processes, indicating that soil bacteria play an essential, but unknown role in element cycling in this environment. Furthermore, under biotic, redox cycling conditions, the rapid (days to months) transformation of meta-stable minerals to stable minerals, which concomitantly sequesters trace contaminants could be possible3. Thus, understanding the microbial ecology, and particularly the identification of bacteria capable of iron-sulphur transformations, is an essential step towards exploiting the microbial community to remediate these environments.
Microbial ecology of acid sulfate soils and sulfidic drain sediments
The microbial ecology was determined using pyrosequencing from samples collected from the model coastal acid sulfate soil site at Blacks Drain, Tweed Valley, NSW, and Australia. The sampling strategy is fully detailed elsewhere5. This site is considered to be a model setting as it is a pollution hotspot (contaminating adjacent waterways with acidity, Al-Aluminum, Mn-Manganese and Fe-iron) has undergone detailed geochemical characterizations of the soils and sediments over the past decade5.
The acid sulfate soils and sulfidic drain sediments contained an average 186 phylotypes per sample in comparison to non-pyritic soils, which contain 5-fold higher ecosystem complexity, with an average 1,017 phylotypes per sample6. Ecosystem complexity is controlled by selection pressures that reduce species diversity7. Acid sulfate soils contain a number of selection pressures associated with the seasonal oxidation of the unstable iron minerals; however, in comparison to acid mine drainage environments (pH 2–4) that contain an average of 61 phylotypes per sample8, these soils have a 3-fold higher ecosystem complexity.
A total of 23 phyla were identified, of which five phyla dominate (>90mm) the community composition, with four common to all environments (Proteobacteria, Acidobacteria, Firmicutes and Chloroflexi), and Bacteriodetes as a major component of sulfidic sediments and Actinobacteria a major component of acid sulfate soil field horizons.
The subdivision of the phyla totalled 48 classes. Focusing on the dominant phyla, drain sediments had two-fold higher abundance of Proteobacteria; and were dominated by δ-proteobacteria. All soil horizons contained δ-proteobacteria but were dominated by β-proteobacteria and α-proteobacteria. Splitting the phylum Chloroflexi into classes revealed that drain sediments were dominated by Anaerolineae in comparison to the soils that were dominated by the Chloroflexi class. The phylum Acidobacteria, also showed that the drain sediments were dominated by the Acidobacteria class and soil horizons dominated by Holophagae. The phylum Firmicutes was dominated by the classes Bacilli and Clostridia across both settings.
Interestingly, there was a low abundance of the class Nitrospira, a group that contains acidophilic Fe(II) oxidising bacteria (Leptospirillum sp.). This is the most important phylotype in acid mine biofilms7; is moderately abundant (average 12mm) in acid mine drainage sites8, but has a low abundance in acid sulfate soils at <3mm. The abundance of this group may be determined by pH due to their limited metabolic capacity (derive energy solely from Fe(II) oxidation) thus are adapted to extremely acidic environments (pH <4)8 where competing abiotic Fe(II) oxidation kinetics are very slow.
Iron and sulphur cycling bacteria in acid sulfate soils
A patchy species distribution and a high proportion of unclassified bacteria characterised acid sulfate soils. Interestingly, these soils lacked an abundance of known acid tolerant Fe(II) oxidisers, of which there are 22 known acidophilic Fe(II) oxidising species across four phyla9. Only the Firmicutes, Alicyclobacillus tolerans was detected in these soils. Instead, the microbial community associated with Fe(II) oxidation was the Betaproteobacteria, Sideroxydans lithotrophicus and Sideroxydans paludicola, with a known ability for Fe(II) oxidation over neutrophilic pH range (4–7.5) under micro-aerobic conditions. Another interesting finding was that Chloroflexus was highly abundant in the acid sulfate soil horizons. Little is known about the role of Chloroflexus in iron cycling, but research from microbial mat zones indicate a positive relationship to zones of enriched Fe(II) oxidation10 and the fully sequenced Chloroflexus aurantiacus contains a candidate Fe(II) trafficking protein (ATCC strain 29364).
Iron reduction is a ubiquitous microbiological mechanism11; but only the neutrophile Anaeromyxobacter dehalogenans was abundant in the acid sulfate soil horizon. Substrate based studies offer the potential to discover the largely unknown Fe(III) reducing the community in these soils as carbon substrates differ between acidophiles and neutrophiles. For example, acetate is key to Geobacter sp.12; however, acetate inhibits Fe(III) reduction in acidic conditions and sugars are a key substrate that stimulates Fe(III) reduction by acidophiles13.
Few S-cycling bacteria were found in the acid sulfate soils; furthermore, considering there are 220 bacterial species known to be involved in sulfate reduction14, it was surprising that no sulfate-reducing species were identified at this taxonomic level. This suggests that there is a significant repository of unknown S-cycling bacteria present in these environments.
Iron and sulphur cycling bacteria in sulfidic sediments
Unlike the acid sulfate soils, the sulfidic sediments were reasonably well resolved and a conceptual model of microbially mediated iron cycling and sulfate reduction in the sulfidic drain sediments was developed.
Biogeochemistry of sulfidic drain sediments impacted by acid sulphate soil leachates.
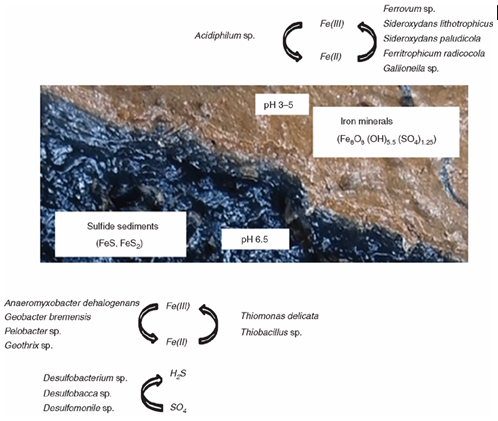
The sulfidic drain sediments contained an abundance of Fe(II) oxidising bacteria, for example, Ferrovum. sp; that oxidises Fe(II) aerobically using CO2. Further, there was an abundance of microbes with capabilities to reduce Fe(III) in the sulfidic sediments which included the acidophile Acidobacterium capsulatum that reduces Fe(III) between pH 2–5, and the neutrophiles Anaeromyxobacter dehalogenans and Geobacter sp. The sulfidic drain sediments contained an abundance of S-compound oxidising bacteria, dominated by Thiomonas delicata. However, similar to the soils, no sulfate reducing species were detected, suggesting that there are many unknown S-cycling bacteria in these environments.
Metal bioaccessibility is correlated to genera abundances
Acid sulfate soil and sulfidic sediments contain highly elevated bioavailable metal(loid) concentrations that are taken up by plants and can be used as biomonitors to isolate pollution hotspots15. A suite of metal(loid)s (Al, As, Cr, Co, Cu, Fe, Mn, Ni and Zn) were positively correlated to genera abundance (Figure 3), indicating that generally genera in acid sulfate environments have high tolerance capabilities to high metal(loid) bioaccessibility, which shape microbial community composition.
Figure 3. Correlations between genera abundance and bioaccessible metal (loid)s including (a) cobalt, (b) nickel, (c) copper and (d) arsenic.
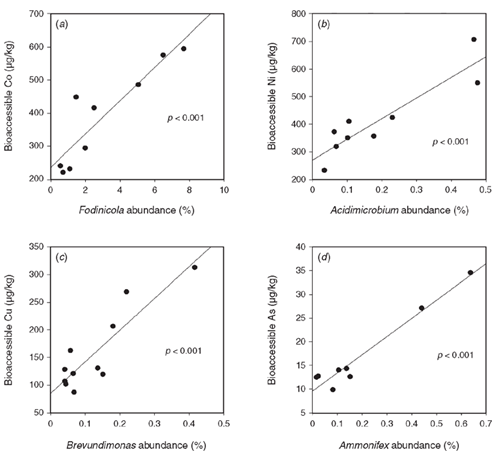
A key finding was the abundance characteristics of the Acidobacteria and Crenarcheota to bioaccessible Manganese concentrations. Recently, these phyla were found to be enriched in Mn-stimulated microcosms and linked to Mn-dependent organic compound oxidation16. Thus, this demonstrates the need for high phylogenetic resolution to investigate environmental factors; because only genus level resolution identified this relationship to soil chemistry.
Understanding the biogeochemistry of acid sulfate soils and sulfidic sediments would transform the management and remediation of these environmentally deleterious sites. The microbial ecology has few parallels to geochemically similar environments, with a microbial community composition including both acidophiles and neutrophiles associated with iron and sulphur cycling. Furthermore, acid sulfate soils and sulfidic sediments house a repository of uncharacterized microbes but abundance patterns correlated to soil chemistry. Taken together, acid sulfate soils may be a model environment that can be used to understand the role environmental conditions play on microbial community compositions, and this information could be used to develop novel biotechnologies.
In the end good land and water management is the process of managing, caring for and developing these resources in both urban and rural settings which may include organic agriculture, reforestation, National Parks, water resource management, creeks, streams and rivers, along with our coastlines and eco-tourism projects not just for this generation but for the generations that follow.
Understanding the biogeochemistry of acid sulphate soils and sulfidic sediments would transform the management and remediation of these environmentally deleterious sites. The microbial ecology has few parallels to geochemically similar environments, with a microbial community composition including both acidophilus and neutrophils associated with iron and sulphur cycling. Furthermore, acid sulphate soils and sulfidic sediments house a repository of uncharacterized microbes but abundance patterns correlated to soil chemistry. Taken together, acid sulphate soils may be a model environment that can be used to understand the role environmental conditions play on microbial community compositions, and this information could be used to develop novel biotechnologies.
In the end good land and water management is the process of managing, caring for and developing these resources in both urban and rural settings which may include organic agriculture, reforestation, National Parks, water resource management, creeks, streams and rivers, along with our coastlines and eco-tourism projects not just for this generation but for the generations that follow.
References
- Fitzpatrick, R.W. et al. (2008) Atlas of Australian acid sulphate soils. In: Inland Acid Sulfate Soil Systems Across Australia (Fitzpatrick, R. W. and Shand, P., eds) vol. 249, pp. 75–89. Perth, CRC LEME.
2. Burton E.D. (2006) Acid-volatile sulfide oxidation in coastal flood plain drains: iron-sulfur cycling and effects on water quality. Environ. Sci. Technol. 40, 1217–1222
| CrossRef |
3. Collins R.N. (2010) Schwertmannite stability in acidified coastal environments. Geochim. Cosmochim. Acta 74, 482–496
| CrossRef |
4. Burton E.D. Johnston S.G. (2012) Impact of silica on the reductive transformation of schwertmannite and the mobilization of arsenic. Geochim. Cosmochim. Acta 96, 134–153
| CrossRef |
5. Stroud J.L. (2012in press.) Metal(loid) bioaccessibility dictates microbial community composition in acid sulfate soil horizons and sulfidic drain sediments. Environ. Sci. Technol., ,
| CrossRef |
6. Lauber C.L. (2009) Pyrosequencing-based assessment of soil pH as a predictor of soil bacterial community structure at the continental scale. Appl. Environ. Microbiol. 75, 5111–5120
| CrossRef |
7. Baker B.J. Banfield J.F. (2003) Microbial communities in acid mine drainage. FEMS Microbiol. Ecol. 44, 139–152
| CrossRef |
8. Kuang J.-L. (2013) Contemporary environmental variation determines microbial diversity patterns in acid mine drainage. ISME J. 7, 1038–1050
| CrossRef |
9. Bonnefoy V. Holmes D.S. (2012) Genomic insights into microbial iron oxidation and iron uptake strategies in extremely acidic environments. Environ. Microbiol. 14, 1597–1611
| CrossRef |
10. Trouwborst R.E. (2007) Biogeochemistry of Fe(II) oxidation in a photosynthetic microbial mat: implications for Precambrian Fe(II) oxidation. Geochim. Cosmochim. Acta 71, 4629–4643
| CrossRef |
11. Weber K.A. (2006) Microorganisms pumping iron: anaerobic microbial iron oxidation and reduction. Nat. Rev. Microbiol. 4, 752–764
| CrossRef |
12. Lovley D.R. Phillips E.J. (1987) Competitive mechanisms for inhibition of sulfate reduction and methane production in the zone of ferric iron reduction in sediments. Appl. Environ. Microbiol. 53, 2636–2641
| CrossRef |
13. Küsel K. (2003) Microbial cycling of iron and sulfur in acidic coal mining lake sediments. Water Air Soil Pollut. Focus 3, 67–90
| CrossRef |
14. Barton L.L. Fauque G.D. (2009) Biochemistry, physiology and biotechnology of sulfate-reducing bacteria. Adv. Appl. Microbiol. 68, 41–98
15. Stroud J.L. Collins R.N. (2014) Improved detection of coastal acid sulfate soil hotspots through biomonitoring of metal(loid) accumulation in water lilies (Nymphaea capensis). Sci. Total Environ. 487, 500–505
16. Beal E.J. (2009) Manganese- and iron-dependent marine methane oxidation. Science 325, 184–187
Jackie Stroud was a post-doc at UNSW. Her research interests are environmental soil science and its applications to sustainable agriculture and bioremediation.
Mike Manefield is an Associate Professor at UNSW. His interests are in environmental microbiology and biotechnology development.
Bioavailability and biodegradation of polycyclic aromatic hydrocarbons Albert L Juhasz
Centre for Environmental Risk Assessment and Remediation (CERAR)
University of South Australia and Cooperative Research Centre for Contamination Assessment and Remediation of the Environment (CRC CARE)
Mawson Lakes, Adelaide
SA 5095, Australia
Further Comments from Readers:
All information is included in good faith and has been thoroughly researched prior to printing. The website or the author does not warrant or guarantee the accuracy of any information on these pages, nor does the website or the author accept any responsibility for any loss arising from the use of the information found within. The views and opinions are strictly those of the author or those members who chose to actively, participate in the contents herein.
“Hi reader, it seems you use The Bible of Botany a lot. That’s great as we have great pleasure in bringing it to you! It’s a little awkward for us to ask, but our first aim is to purchase land approximately 1,600 hectares to link several parcels of N.P. into one at The Pinnacles NSW Australia, but we need your help. We’re not salespeople. We’re amateur botanists who have dedicated over 30 years to saving the environment in a practical way. We depend on donations to reach our goal. If you donate just $5, the price of your coffee this Sunday, We can help to keep the planet alive in a real way and continue to bring you regular updates and features on Australian plants all in one Botanical Bible. Any support is greatly appreciated. Thank you.”
In the spirit of reconciliation we acknowledge the Bundjalung, Gumbaynggirr and Yaegl and all aboriginal nations throughout Australia and their connections to land, sea and community. We pay our respect to their Elders past, present and future for the pleasures we have gained.