Plants play a central role in the sustainability of life on earth. Their function is strongly influenced by their intimate interaction with deleterious and beneficial organisms from the smallest microbes to the largest herbivores.
An integrative approach is needed to understand where the mechanism by which the microbes and/or insects interact with the plant and subsequently what affects the plant has in the relationship can take many directions. The greater the number and variety of individual microbes and insects that are reacting with the plant at any one time makes any conclusion more hypothetical than conclusive. This is further compounded as time goes by as the affects of earlier interactions may affect the results of future interactions.
Most studies on plant mediated interactions in the past have focused on organisms that share a common domain, mainly above ground. During the last decade, however, numerous ecological studies have revealed that the plant interactions with attackers or beneficial organisms below ground can strongly affect the interactions that occur above ground and vice versa. New research into the physiological mechanisms of plants reveal that induced plant responses occur as a result of responses to herbivorous insects and microbial pathogens, as well as to beneficial organisms such as root symbiotic relationships. These are all regulated by a network of a relatively few signaling pathways that can cross the root shoot divide.
Most mechanical studies mainly focus on responses to a single attacker above ground or a root symbiotic relationship below ground.
Even at its simplest the organic farmer or home gardener is able to capitalize on these results.
In the field, however, plants are constantly confronted with a complex community of competing foliages, roots, soil dwelling attackers and beneficial organisms with a near finite resource. Now there is an increasing interest to explore plant immune responses in this context. Added to this, is the variability of the soils themselves with their different physical and chemical characteristics.
Beneficial Microbes are able to alter
Responses to Multiple Stresses
The symbiotic relationships with beneficial microorganisms result in important changes in the plant physiology that are frequently related to the plants growth, development and health. They also have an important impact on the plant’s ability to cope with stress, heat, water transpiration, disease and functions. This induction to tolerance or resistance to stresses may explain the perpetuation of plant microbe symbioses in conditions where there are no nutritional benefits for the plant.
Beneficial Microbes Can Induce Resistance
or Susceptibility to Herbivores
In addition to locally suppressing pathogens via competition or via the production of antibiotics and siderophores, beneficial soil borne microbes can enhance plant resistance against a wide range of pathogens and herbivores in systemic plant tissues. The mechanisms regulating the plant interaction with beneficial microbes, and those underlying the effect of these interactions on organisms deleterious for the plants health and growth, are shared by taxonomically different groups of beneficial microbes.
Beneficial Microbes Induce Tolerance to Abiotic Stress
Beneficial microbes may also induce host tolerance to abiotic stresses in the soil, such as the presence of heavy metals, the lack of nutrients, drought and salinity as well as to above ground abiotic stresses such as high CO2, heat or light stress. Environmental conditions in the natural ecosystem of particular plants may influence whether mutualism increases or decreases tolerance to abiotic stresses. For instance, colonization by the endophyte Neotyphodium increased regrowth after drought in a Lolium perenne genotype from a dry area, but not in a genotype from a wet area (Hesse et al. 2004). Thus, when searching for microbial strains to induce tolerance to abiotic stress, it is important to search under the conditions where both plant and microbe are naturally experiencing the stress and compare it to plants that are not experience the stress at the same time.
One clear effect of the beneficial soil microbiota is the increase in root growth and the alteration of root architecture. The improvement of the root system may enhance tolerance to nutrient deficiencies and drought by increasing the volume of soil to be explored in the search for nutrients and water. It has been noted by the author that plants growing in nutrient rich medium develop a smaller root system than those growing in a nutrient poor medium. Interestingly, these root modifications are partly mediated by changes in phytohormone levels associated with the microbial colonization. Altered hormone levels may explain the differential response of symbiotic plants to stress. For example, mycorrhizal plants usually have altered Abscisic Acid (ABA) levels, and ABA is essential for the enhanced tolerance to abiotic stress that is induced by mycorrhizal fungi (Aroca et al. 2008). Rhizobacteria may also alter phytohormone levels under stress conditions. For example, ET production increases under some abiotic stresses such as drought, reducing plant growth. Under drought stress rhizobacteria can produce ACC deaminases that degrade the ET precursor ACC, thereby decreasing ET content and restoring normal plant growth (Glick et al. 2007). Gibberellins are chemical substances that were isolated first from a fungus. Increased levels of ABA acts as an inhibitor preventing seeds from germinating and buds from developing during cold weather, drought or at other times of stress.
Plant Beneficial Microbe Symbioses in a
Changing Environment
For a beneficial microbe to enhance plant tolerance to a particular stress, the plant microbe symbiosis needs to be functional under such stress conditions. Abiotic stresses can affect beneficial microbes directly, or indirectly via changes in the host plant physiology and/or root exudation. Several studies have recently reviewed the effects of climate change on the interactions of plants with beneficial microbes The majority of plants in these studies showed that elevated CO2 levels had a positive influence on the abundance of mycorrhizal fungi, whereas the effects on rhizobacteria and endophytes were more variable. In contrast, drought and soil salinity appear to decrease the abundance of beneficial microbes. The effects of increased temperatures have been highly variable for all groups of microbes, but for mycorrhizal fungi most studies suggest an increase in their abundance. Soil pH is also an important determinant not only of microbial populations but also of microbial activity, affecting, for example, symbiotic efficiency in mycorrhizae. Collectively, these reviews show that abiotic stresses can modify the abundance and composition of soil microbial communities and, consequently, their effects on the plants. (Compant, van der Heijden & Sessitsch 2010; Pritchard 2011; Aroca et al. 2013; Chakraborty, Pangga & Roper 2012; Hayman & Tavares 1985).
A well established symbiosis with mycorrhizal fungi or rhizobacteria is the first prerequisite to observe a beneficial effect of microbes on plants, and in some cases, a threshold of colonization is required. Therefore, abiotic stresses that affect the microbial colonization of the roots may impair the microbial induction of ISR. However, a better microbial colonization may not necessarily result in a stronger induced resistance. For instance, a rise in CO2 increases ryegrass (Lolium perenne) colonization by the endophyte Neotyphodium lolii but decreases the concentration of alkaloids, which are the toxic compounds that mediate the resistance conferred by these endophytes. Similarly, we would expect plant fitness to be enhanced when environmental changes lead to an increase in symbiotic abundance, but this seems to occur only when the limiting factor for plant growth is the same that the symbiosis is alleviating (Compant, van der Heijden & Sessitsch 2010).
Changes in the microbial community composition will affect herbivores, because combinations of certain strains or species of bacteria and mycorrhiza have synergistic effects whereas others have antagonistic effects (Gange, Brown & Aplin 2003; Saravanakumar et al. 2008). The importance of the microbial community composition can also be observed for the interactions of plants with members of the third trophic level, for example parasitoids. Depending on the combination of mycorrhizal species colonizing Leucanthemum vulgare plants, parasitism of the leaf miner Chromatomyia syngenesiae by the parasitoid Diglyphus isaea was enhanced or reduced (Gange, Brown & Aplin 2003). Therefore, understanding how environmental conditions affect microbial communities may shed some light on how herbivore communities respond to environmental changes.
Plant Microbe Insect Interactions in a Changing Environment
While abiotic stresses are predicted to increase with climate change, the interactions of plants with microbes and with insects are also likely to increase or decrease conversely with different actions and reactions. Will abiotic factors affect the plant’s mediated effects of microbes on herbivores?
Some research has been carried out on beneficial fungi; mycorrhizae and other endophytes but nothing to date has been researched for plant growth promoting rhizobacteria or nodulating rhizobia, however, there is some evidence surfacing that abiotic stress may strengthen certain microbial effects on plant resistance. Although a few studies have explored how stresses such as salinity (Younginger, Barnouti & Moon 2009), ozone (Manninen et al. 2000) and CO2 (Marks & Lincoln 1996) affect plant–microbe–insect interactions. To date most research on these topics has been centered on the effects of nutrient levels and drought on growth.
Soil nutrient content plays an important role in plant–insect interactions with mycorrhizal fungi and fungal endophytes, with converse effects between the two groups of microbes. Mycorrhizal fungi can have positive or negative effects on herbivores, but such effects were not observed or were minimal when the soil is supplemented with additional phosphorous (Borowicz 1997; Gange, Bower & Brown 1999) or nitrogen (Gange & Nice 1997; Manninen, Holopainen & Holopainen 1998).
There was a negative effect of fungal endophytes on herbivore performance was strengthened by the addition of nitrogen or an NPK fertilizers, probably as a result of promoting increased synthesis by the endophyte of nitrogen based alkaloids that are toxic for the herbivores. (Lehtonen, Helander & Saikkonen 2005; Vesterlund et al. 2011).
The relevance of drought as regulator for plant–microbe–insect interactions to date has also been demonstrated for fungal endophytes (Vidal 1996; Bultman & Bell 2003; Miranda, Omacini & Chaneton 2011; Vesterlund et al. 2011; Yule, Woolley & Rudgers 2011). Most of these studies evaluated the effects on phloem feeders, (Bultman & Bell 2003 and Vesterlund et al. 2011) while for other herbivores, which are generally negatively affected by endophyte, infection only under certain level of drought stress (Bultman & Bell 2003). For instance, the negative effect of the endophyte Neotyphodium coenophialu on the aphid Rhopalosiphum padi was only present when plants recovered from the stress but not when the plants were continuously under water stress (Bultman & Bell 2003). Such effects may be related to the concentration of loline alkaloids, toxic for aphids, which was found to be higher in previously stressed plants (Wilkinson et al. 2000). It is not yet known whether drought affects plant–insect interactions with microbes that do not produce alkaloids, and how herbivores other than phloem feeders would respond remains to be elucidated.
Even less attention has been paid to the modification of multitrophic interactions between microbes, plants, herbivores and their natural enemies by abiotic stresses. To my knowledge, the first study addressing this aspect was recently published by Yule, Woolley & Rudgers 2011. Their research was carried out with the endophyte Neotyphodium PauTG-1 colonizing the grass Poa autumnalis. It assessed the effects on the aphid Rhopalosiphum padi and on its parasitoid Aphelinus sp. Under field conditions, a moderate water stress treatment strengthened the positive effect of endophytes on plant biomass and parasitoid incidence subsided when normal watering regimes were in place. They noted that when the plants were subjected to water stress it did not affect plant biomass of the endophyte free plants. Their results suggest that a plants response to moderate abiotic stress may affect plant–microbe–insect interactions, independent of the effects on individual plant growth.
Are Beneficial microbes Recruited under Favourable Conditions?
Symbiotic microbes are beneficial for plants mainly when plants need help, and this need is determined, at least in part, by the occurrence of biotic and abiotic stresses. Beneficial microbes are initially treated as invasive organisms with the plant responding accordingly as it would to any invasive organism. As the plant recognizes the organism the defensive responses are withdrawn and a symbiotic relationship is entered into. (Pozo & Azcon-Aguilar 2007; Zamioudis & Pieterse 2012). What triggers the withdrawal of the defenceses is unknown but it is suspected that it may be related to the plants needs not beig satisfied. It is well documented that several symbionts only benefit their host plants under limiting conditions, where the benefit may outweigh the costs of maintaining the symbiotic relationship. (Hoeksema et al. 2010) For example; Breuillin et al. 2010, researched and proved that the main benefit of a mycorrhizal symbiotic relationship that an improved phosphorous acquisition, under low phosphate conditions, the plants he researched promoted an interaction and triggered a symbiotic program. Contrary though when phosphate levels were adequate the plants inhibited the activation of any symbiotic genes and reduce mycorrhizal colonization of the roots.
As previously discussed, beneficial microbes can also play a crucial role in plant survival and/or fitness by triggering defence mechanisms. It therefore stands to reason considering Breuillin et al. 2010 research that microbial symbioses are promoted whenever environmental conditions are all but ideal to improve stress resistance.
Interestingly, studies with disease suppressive soils have shown that a strong disease outbreak is required before any suppression is activated by the plant which Mendes et al. 2011 research indicated supports the idea of beneficial microbes being incorported into any defense strategy after the onset of invasion.
There is evidence that upon above ground herbivory, mycorrhizal colonization can increase (Kula, Hartnett & Wilson 2005), although it may revert after intense removal of the foliage. Recently, the first proof of rhizobacteria recruitment upon above ground herbivory has been published. The mechanisms that explain how the microbe-plant symbiosis is enhanced after the plants perceive a certain stress are only now starting to be uncovered. Our methods of farming by cutting clover (Haifa trifoliata) back hard with the onset of bud swell in citrus between 1992 to 2008 meant we never needed to supplement the orchard with nitrogenous fertilizers and supports Gehring & Bennett 2009 and Yang et al. 2011; Lee, Lee & Ryu 2012.
At the time without a laboratory and the specialized equipment needed to carry out any in depth research I could only hypothesize on what was happening beneath the soil and above the ground. Leaf analysis however did confirm that better than adequate supplies of nitrogen were present and consistent throughout the orchard. My hypothesis of what happened is certainlyindicative of what was happening below the soil surface and has now been substantially proven by the above mentioned researchers and their research which have been recently released.
Advances have shown that plants display certain characteristics below the ground via the production of certain compounds that attract enemies of root herbivores (Rasmann et al. 2005) or that enhance the root colonization by beneficial microbes (Rudrappa et al. 2008; Lopez-Raez, Pozo & Garcia-Garrido 2011; Neal et al. 2012). For instance, pathogen attack on Arabidopsis leaves increased the root exudation of malic acid and this led to the recruitment of the PGPR Bacillus subtilis (Rudrappa et al. 2008). Phytohormones may also play a role. For example, an increase in JA after repeated mechanical damage above the ground that partially simulates herbivory damage seems to enhance mycorrhizal colonization of roots in Medicago truncatula. Our methods of farming by cutting clover (Haifa trifoliata) back hard with the onset of bud swell in citrus between 1992 to 2008 strongly supports Landgraf, Schaarschmidt & Hause 2012 research.
Help may be also be forthcoming under abiotic stress conditions. Research about a new group of plant hormones, strigolactones, showed that plants increase their production under phosphate limiting conditions. Interestingly, these hormones have several functions. In the rhizosphere as they are key signalling molecules in the establishment of the mycorrhizal symbiosis, ultimately improving the plant’s capacity to acquire phosphorous. Within the plant, they regulate shoot branching and root architecture, which may indirectly affect plant tolerance to abiotic stress (Lopez-Raez, Pozo & Garcia-Garrido 2011). Under salt stress, mycorrhizal plants increase the production of strigolactones (Aroca et al. 2013). The synthesis of these hormones is regulated by ABA. (Lopez-Raez et al. 2010)
It is now possible to hypothesize that abiotic stress may increase strigolactone production to promote the mycorrhizal symbiosis and alleviate stress. As plants in nature are simultaneously affected by biotic and abiotic stresses, it would not be surprising if the production of compounds that favour beneficial plant–microbe symbioses are further enhanced when contolling both biotic and abiotic stresses.
Plant Insect Interactions in a Changing Environment
For over 350 million years plants and insects have coexisted in a perpetual battle of survival one pitted against the other trying to gain the upper hand. This perpetual battle of defenses has seen develop a diverse range of physical barriers, chemical exchanges and insect harbouring techniques to outwit the voraciousness of their herbaceous insect opponents. Their techniques to outsmart their opponents has largely been successful as over 90mm of the insects have evolved, dine on just one or a few closely related species or genre.
Apart from physical barriers one mechanism insects use to deter insect attack or disease is known as effectors. These may work against the plants defence system or specific effectors maybe required to promote compatible insect plant interrelationships.
Herbivore feeding mechanisms vary as much as the plants defence mechanisms.
A pointed case is clover; white Haifa, is cut as the apical buds on Citrus are expanding, the clover will relinquish some of the nitrogen fixing bacteria die releasing the soluble nitrogen into the soil as it is not required by the plants at this stage. As a result the Citrus needing additional nitrogen at this stage is quick to take the discarded nitrogen released from the clover up. Cutting the clover, in reality to the clover, is similar as being grazed upon by a larger herbivore.
Caterpillars are chewing insects that release many different elicitors from the plant that can induce defense responses as distinguishable from mechanical damage or the grazing of a large herbivore.
The gall wasps and Aphids use different elicitors to stimulate galls, secretion flow or prevent the release of toxins or induce sugary sap flow.
These sap sucking insects of the Hemiptera species first started to evolved and diverge from the Holometabola around 320million years ago. The hemiptera insects are distinguished from the Holometabola in that their moth parts which have evolved into distinct stylets for piercing and sucking while displaying active suppression of plant defense responses.
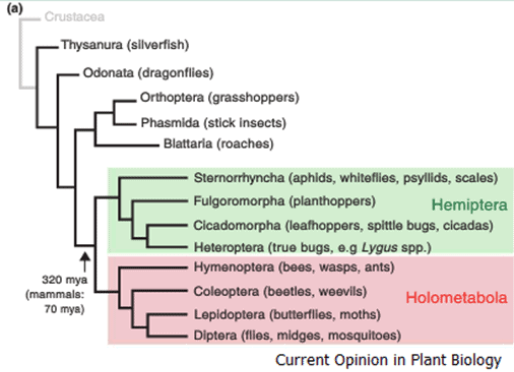
Here aphids often initiate probing of the leaf surface immediately on landing. The stylet penetration of the epidermis cell wall and membrane injection of saliva and ingestion of sugary compounds takes less than a minute. In a compatible interaction the Aphids stylet will extend into the phloem or xylem to establish a long term feeding station. The aphids inject two main types of saliva. The first is a gel like substance that covers and protects the stylet from the plants defence systems while the second a watery compound is secreted into the plant cells during salvitation.
The Gel and saliva both contain proteins that function as effectors to induce or suppress plant responses. The induction of animal salivas can also transmit viruses and pathogens which can result in Plant Trigger Immunity Responses (PTI) from the plant.
A successful pathogen or virus can also deploy effectors that can suppress PTI resulting in a compatible interaction. The plant will enter into a phase two reactions on recognition of the virus or pathogens effectors resulting in Effector Triggered Immunity (ETI). The virus or pathogen may avoid the ETI by diversifying or shedding the effectors’ gene or suppressing the ETI by releasing other/new effectors which may set off another round of plant PTI responses.
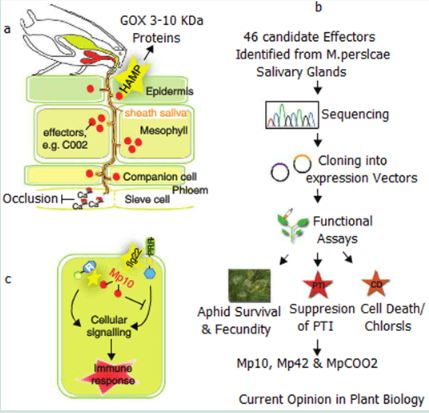
Herbivore Associated Molecular Patterns (HAMP) are key components of insects and are considered to exhibit similar activities as Plant Associated molecular Patterns (PAMP) in invoking the first line of defence in plants. The following effectors stimulate elicit plant defences or HAMP. They include modified Lipids such as Fatty Acid-Amino Acids, Conjugates (FAC), Glucose oxidase, Beta-glucosidase and inceptins from lepidopterans and sulphur containing Fatty Acid calciferrins from grasshoppers.
At the same time the Gene G002 is being advanced more rapidly in Aphids than any other group to promote plant insect reactions in favour of the Aphid. It must be noted that plants can detect and recognize elicitors in insect oral secretions and can also detect minute elicitors even during egg depositation.
The evidence that insects secrete proteins in their saliva came from Musser et al in his works that the caterpillar Helicoverpa zea secreted the enzyme glucose oxidase (GOX) into its host to suppress the production of nicotine and resistance in Nicotiniana tabacum. It has since be found that GOX is commonly found in many herbaceous insects that consume green matter.
Further the saliva of Aphids can suppress the ability of the host to set up clogging agents or the sieve elements so that feeding may continue unheeded. This occlusion of elements depends on the influx of Ca2+ into the sieve element lumen. Aphid saliva contains calcium binding proteins which prevent the calcium proteins from binding thus suppresses the plants defence system.
Studies done by Mutti et al identified the salivary gland gene G002 from Acyrthosiphon pisum as the gene which encodes a secreted protein which when injected into the host during feeding. Blocking this gene in Aphids has resulted in Aphids altering their feeding behaviour. Phylogenetic analysis has shown that this gene is more rapidly evolving in Aphids than any other insect.
Defence responses from both insects and plants involve multiple Herbivore Associated Molecular Patterns (HAMP) triggered immunity (HTI) and ETI which is also applicable to plant- insect interactions and the targeting of specific effectors in response to the others effectors and counter effectors. Once an insect is unable to secrete effectors that suppress the plants HTI the insect stops any further feeding.
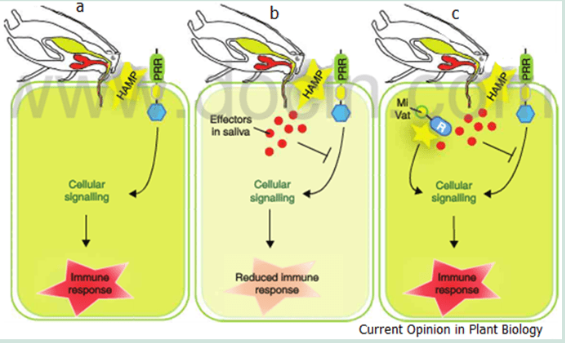
Remember:
- Exciting discoveries in the last decade indicate that plant responses to microbes and insects share important similarities and common regulators, and in recent years, these research fields have been frequently connected. However, the interconnection between these two research fields has mainly been based on individual microbe specie reactions or herbivore specie reactions. There is still a big gap on how microbes affect insects at the community level, including herbivores from different feeding guilds and insects of higher trophic levels to the more advanced vertebrae carnivores.
2. A trophic cascade experience could easily be explained with a caterpillar a bird and a thistle. The insect in this case and the bird are directly responsible for the direction and colour of the flowers. This is where the predator determines the traits of the herbivore or the herbivore determines the traits of a plant through coevolution.
3. If a certain caterpillar is eating a thistle and they preferred to eat the thistles with the white flowers, the thistle would eventually end up with only producing coloured flowers.
The traits of the coloured flowers whether they are toxic, insipid or foul tasting will predominate over the white flowering plants.
4. f the birds only fed on the caterpillars that consumed the white flowers, then the white flowers may take over as the only moths not eaten by the birds are those eating the purple flowers. The plants with white flowers may not take over as they still have the more desirable affects for being eaten.
5. The coloured flowering thistles will develop stronger chemicals or defences against the moths still eating them.
Such a scenario depends entirely on the predatory bird. If something were to happen to the bird (Disease, predator or land clearing affecting its habitat) then an explosion in numbers of the moths eating the white flower flowers will occur with the subsequent decrease in the number of white flowers again giving the coloured flowering thistles the upper hand.
6. Plants are members of complex communities formed by multiple species of insects and microbes that may be detrimental or beneficial for the plant and be located above or below the ground. Unfortunately, plant–microbe and plant–insect interactions have long been studied in isolation in the past. The realization now that they need to be studied as a complex evolving and contracting system of simultaneous affects of biotic and abiotic stresses and ideals.
Further Comments from Readers:
All information is included in good faith and has been thoroughly researched prior to printing. The website or the author does not warrant or guarantee the accuracy of any information on these pages, nor does the website or the author accept any responsibility for any loss arising from the use of the information found within. The views and opinions are strictly those of the author or those members who chose to actively, participate in the contents herein.
“Hi reader, it seems you use The Bible of Botany a lot. That’s great as we have great pleasure in bringing it to you! It’s a little awkward for us to ask, but our first aim is to purchase land approximately 1,600 hectares to link several parcels of N.P. into one at The Pinnacles NSW Australia, but we need your help. We’re not salespeople. We’re amateur botanists who have dedicated over 30 years to saving the environment in a practical way. We depend on donations to reach our goal. If you donate just $5, the price of your coffee this Sunday, We can help to keep the planet alive in a real way and continue to bring you regular updates and features on Australian plants all in one Botanical Bible. Any support is greatly appreciated. Thank you.”
In the spirit of reconciliation we acknowledge the Bundjalung, Gumbaynggirr and Yaegl and all aboriginal nations throughout Australia and their connections to land, sea and community. We pay our respect to their Elders past, present and future for the pleasures we have gained.